KEY POINTS
• Athletes with spinal cord injury (SCI) often have reduced resting metabolic rate (RMR) and increased body fat due to lower active muscle mass and decreased metabolic rate, necessitating dietary adjustments to prevent nutrient deficiencies.
• Athletes with amputations (AMP) experience a reduction in fat-free mass (FFM), leading to a decreased RMR, particularly in those with lower- leg amputations.
• Athletes with cerebral palsy (CP) often face higher energy costs due to less efficient movements and muscle stiffness.
• Limited visual feedback in athletes with visual impairments (VI) impacts motor learning and pacing, which can alter performance timing and pacing efficiency, especially in sports like swimming. Athletes with VI may lack visual feedback to gauge hydration and energy status.
• There is no "one-size-fits-all" approach to assessing body composition in Para athletes due to the diverse impacts of impairments, which result in unique distributions of muscle and fat across different impairment types and activity levels. This requires tailored assessment methods to provide accurate and meaningful data.
INTRODUCTION
The Paralympics provide a unique sporting environment for athletes with various physical, visual and intellectual impairments, classified into ten eligible impairment types that must be permanent in nature (Table 1). Each impairment presents specific challenges that coaches, sports practitioners and athletes must address to optimize training, performance and nutrition. This sports science exchange (SSE) article focuses on key considerations for athletes with spinal cord injury (SCI), amputations (AMP), cerebral palsy (CP) and visual impairments (VI), whose performance and physiological adaptations differ significantly from non-disabled athletes. This SSE article aims to guide sports science practitioners new to Para sports.
SPINAL CORD INJURY
Research in sports like wheelchair racing, wheelchair rugby, wheelchair basketball and wheelchair tennis, has highlighted physiological differences between athletes with SCI and non-disabled athletes (Bhambhani, 2002; Goosey-Tolfrey & Leicht, 2013). These differences include a reduced active muscle mass, lower stroke volume due to impaired muscle pumps in the lower limbs and potentially reduced maximum heart rate (Paulson & Goosey-Tolfrey, 2017). The extent of these physiological impacts depends on the lesion level and completeness: high, complete lesions result in greater impairment, while lower, incomplete lesions result in physiological responses more comparable to non-disabled athletes (Bhambhani, 2002).
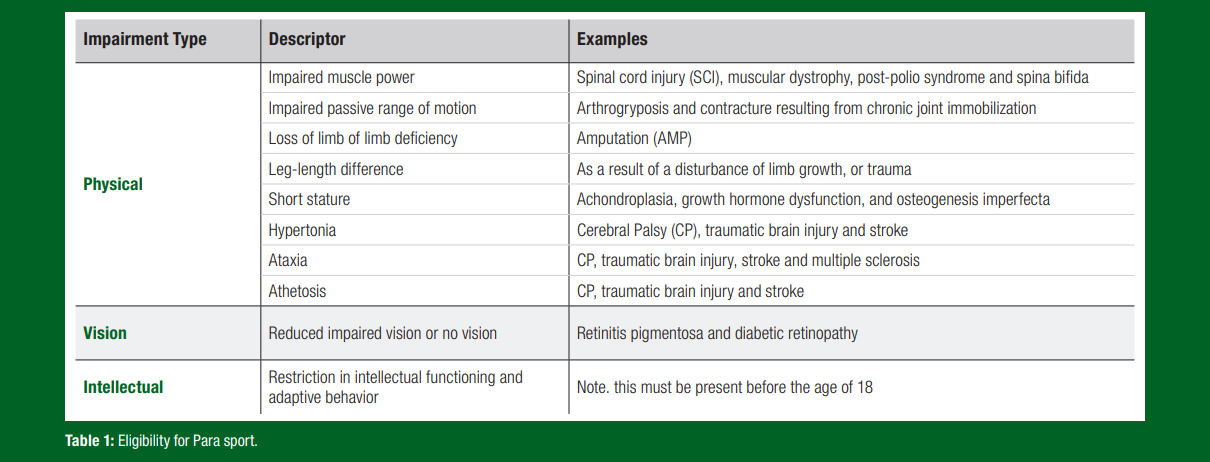
Segmental analyses from dual-energy X-ray absorptiometry (DXA) show regional fat and bone mineral density (BMD) differences. Wheelchair athletes display greater BMD, more lean mass and a lower percent fat in their arms, with the reverse of the legs when compared to non-disabled individuals (Sutton et al., 2009). Comparing different sports, the lowest fat mass was found in paracycling athletes, and the reader is directed to Flueck (2020) for further information.
Athletes with a SCI also experience mechanical constraints, such as impaired trunk function due to muscle paralysis, which can reduce the effectiveness of coughing and respiratory function (Brown et al., 2006). The decentralization of the autonomic nervous system in athletes with SCI can lead to immunoendocrine deficiencies (Nash, 2000). Regular use of a wheelchair for daily mobility - a less efficient form of movement - places additional demands on the upper limbs during both daily activities and training (Webborn & Van de Vliet, 2012). Additionally, lower active muscle mass and reduced metabolic rate diminish total energy expenditure, often necessitating dietary adjustments to avoid nutrient deficiencies. This lower intake can lead to macronutrient and micronutrient deficits, which are compounded by gastrointestinal (GI) complications, such as neurogenic bladder and bowel issues, GI dysbiosis and slower transit times (Abel et al., 2017). These issues further affect nutrient absorption and energy balance, increasing the risk of conditions like urinary tract infections or pressure ulcers that may disrupt training and impact performance.
Athletes with SCI also face challenges in thermoregulation, as disrupted afferent signaling to the thermoregulatory center impairs vasomotor control and sweating below the lesion level (Grossmann et al., 2021; O’Brien et al., 2022; Goosey-Tolfrey & O’Brien, 2024). This is compounded by the loss of venous muscle pumps, which normally help redistribute blood from paralyzed areas (Hopman et al., 1992). SCI-related muscle paralysis can lead to increased body fat levels and greater peripheral fatigue, which influences the perception of exertion during or after exercise as peripheral fatigue cues dominate over central ones (Iturricastillo et al., 2016). Finally, the distinct physiological demands across wheelchair sports contribute to variations in body composition among athletes with SCI, as seen in hand cyclists who typically have lower fat-free mass (FFM) compared to non-disabled athletes (Goosey-Tolfrey & Leicht, 2013).
AMPUTATIONS
Athletes with AMP may compete in either wheelchair or ambulant classes based on the extent of their impairment. Due to amputation, these athletes experience a reduction in body mass, body surface area and active muscle mass, which varies based on the amputation level. This difference is evident in DXA comparisons with non-disabled individuals (Sherk et al., 2010). Lower-leg AMP, for instance, lead to reduced resting metabolic rate (RMR) due to a decrease in FFM, though specific data on Para athletes is limited (Scaramella et al., 2017).
Ambulant athletes with AMP often exhibit movement inefficiencies like those observed in athletes with cerebral palsy (CP), which will be discussed next (Blauwet et al., 2017; Ward & Meyers, 1995). Energy expenditure (EE) during ambulation at a consistent workload is significantly higher for athletes with AMP than for non-disabled individuals, and the metabolic cost increases with the level of amputation (Ward & Meyers, 1995). Notably, above-knee AMP experience even higher energy costs in activities like walking (Menglkoch et al., 2017). While limited data exists on EE in cycling among athletes with AMP, studies indicate altered gross efficiency and pacing compared to non- disabled cyclists, leading to considerable differences in performance times (Scaramella et al., 2017). However, in certain sports – particularly those emphasizing upper-body performance or where reduced weight can offer a competitive advantage (e.g., wheelchair racing), athletes with AMP may experience lower EE compared to non-disabled athletes, highlighting the complex interaction between impairment, sport-specific demands and EE.
A unique challenge for athletes with AMP is a reduced body surface area relative to their volume, due to missing limbs, which limits evaporative and convective heat loss, leading to greater heat retention (Webborn, 1996). Prosthetic socket liners and the prostheses themselves add insulation, impeding heat dissipation and increasing the risk of skin injuries on the stump (Klute et al., 2007). Additionally, skin grafts in these areas limit sweating and vasodilation further compromising heat regulation (Crandall & Davis, 2010). The contact site of the prosthesis poses a risk for pressure sores, discomfort and infection, which require careful management of training volume, prosthesis fit, nutrition and infection prophylaxis (Scaramella et al., 2017). This issue is exacerbated during large-scale events like the Paralympics, where distances between the Paralympic village, transport mall and dining hall are significantly longer compared to a home environment. Practical adjustments are required to avoid such complications.
CEREBRAL PALSY
Athletes with CP display significant movement inefficiencies largely due to movement dysfunctions stemming from damage in areas of the brain (Blauwet et al., 2017). Specifically, the presence of athetosis, spasticity or ataxia, may all limit muscular coordination and increase the energy cost of movements (Blauwet et al., 2017). Studies, albeit in children, show that movement inefficiencies lead to elevated metabolic heat production for an absolute external workload (Maltais et al., 2004). High muscle tone, spasticity and reduced ability to stretch can limit control over movements, impair venous return via muscle pumps and negatively impacts on blood flow distribution exacerbating fatigue (Kloyiam et al., 2011). The internal workload required to achieve a set intensity is thus significantly greater in athletes with CP, increasing their susceptibility to excessive overload.
Total energy expenditure (TEE) in individuals with CP can be highly variable, influenced by factors like sex, ambulation status and body composition, with DXA showing variations based on ambulation status (Scaramella et al., 2017). Nutritional assessment, including oral-motor function, body composition and energy balance, is essential to support the health and performance of athletes with CP (Flueck, 2020).
VISUAL IMPAIRMENT
While athletes with VI are generally physiologically similar to non- disabled athletes, their impairment requires specific performance considerations, though RMR is not expected to be substantially reduced (Broad, 2019). Limited visual feedback impacts motor learning, proprioception and the development of effective techniques (e.g., in swimming or running) to manage training loads (Taylor et al., 2016; Magno e Silva et al., 2013). Restricted visual input also affects pacing; for instance, swimmers with a VI demonstrate altered pacing in 400 m events, likely due to reduced pace awareness (Taylor et al., 2016). Moreover, athletes with VI face challenges in self-monitoring hydration status, as they cannot assess urine color or volume as easily (Webborn & Van de Vliet, 2012). Moreover, athletes with albinism also face heightened risk of sunburn in high-radiant environments due to low skin pigmentation (Stephenson & Goosey-Tolfrey, 2020).
ASSESSMENT OF BODY COMPOSITION IN PARA ATHLETES
A variety of techniques are available to assess body composition in athletic populations. These include field-based methods, e.g., skinfold calipers and those that require minimal equipment, such as bioelectrical impedance analysis (BIA), through to laboratory-based techniques, e.g., hydrostatic weighing, air displacement plethysmography and DXA. The suitability of these tools to monitor changes in both fat mass and FFM across the competitive season present unique challenges when applied to wheelchair versus ambulant para-athletes. Techniques such as body mass index (BMI), waist circumference and BIA often rely on assumptions about muscle and fat distribution that may not hold true for athletes with SCI or other disabilities. For wheelchair athletes, who experience altered muscle mass distribution and body composition compared to their ambulant counterparts, traditional metrics can lead to inaccurate interpretations and assessments. This highlights the need for tailored approaches and careful consideration of the limitations and potential biases of existing body composition tools to ensure reliable and valid assessments in this specialized athletic population. It is beyond the scope of this article to provide further in-depth discussion and so the reader is guided to Slater and Goosey-Tolfrey (2019).
ENERGY EXPENDITURE VARIATION BY SPORT AND TYPE OF IMPAIRMENT
Energy expenditure (EE) in Para sports not only differs significantly based on the sport's demands but also on the type and severeness of impairment outlined earlier in this SSE article. In this context, the various components of EE such as total daily EE (TDEE), RMR and EE during physical activity and exercise must be considered. This fact is underlined by a recent study (Weijer et al., 2024) about energy requirements in Para sports, which reported a wide range of TDEE from 2322 ± 340 kcal·d−1 (wheelchair basketball) to 3607 ± 1001 kcal·d−1 (para cycling). Absolute RMR ranged from 1258 ± 283 kcal·d−1 (Nordic skiing) to 1683 ± 276 kcal·d−1 (para cycling), whereas no significant differences between sport disciplines were found, if relative RMR (kcal·kg−1, FFM·d−1) was reported. Considering energetic aspects, such as the amount and composition of macronutrient intake, it is crucial in Para sports to ensure adequate body mass and composition, optimize exercise performance and address health issues like low energy availability (LEA).
EE and SCI
Wheelchair sports like table tennis and fencing show the lowest EE levels among athletes with SCI (Price, 2010). These sports involve relatively static movements, often with fewer dynamic muscle actions than their non-disabled counterparts. Similarly, wheelchair rugby also records lower EE, largely because athletes in this sport are predominantly those with tetraplegia, where limitations in muscle mass and motor function restrict energy output.
In contrast, wheelchair tennis and basketball demand higher energy output due to their greater physical demands. Interestingly, Croft et al. (2010) observed that elite wheelchair tennis and basketball players exhibit considerably higher EE compared to their recreational or national-level peers, highlighting the impact of training intensity and athletic level on EE in athletes with SCI. Table 2 provides an overview on EE for different wheelchair sports. It becomes obvious, that values vary considerably based on sports discipline and level of the SCI but are often far below values found in non-disabled athletes, where EE of up to 20 kcal·min-1 were documented.
One of the primary reasons for lower EE in athletes with SCI athletes compared to non-disabled athletes is the reduced muscle mass actively engaged during both everyday movements and sporting activities. This limitation is due to muscle atrophy in the lower limbs from restricted mobility, especially in those relying on wheelchairs, which significantly lowers their baseline energy requirements. Another crucial factor influencing EE is the sympathetic nervous system (SNS) activity, particularly its influence on the body's metabolic response to exercise, involving the release of catecholamines like adrenaline and noradrenaline. Research by Schmid et al. (1998) and Steinberg et al. (2000) demonstrates that individuals with SCI, particularly those with higher-level injuries (e.g., T1-T5), often have a reduced catecholamine response. This blunted SNS response is especially pronounced in athletes with quadriplegia and high-level paraplegia, leading to a decreased capacity for efficient energy use, particularly from carbohydrate and fat stores. The lower SNS response further impacts energy metabolism and contributes to the overall reduction in EE observed in athletes with SCI. Consequently, the energy requirements and recommendations for carbohydrate (Ruettimann et al., 2021) and protein intake (Flueck and Parnell, 2021) of SCI athletes vary widely, necessitating individualized training and nutrition approaches to support their athletic performance. This is not only important to avoid unnecessary gains in body mass but also to avoid the occurrence of LEA, which may lead to relative energy deficiency in sports (RED-S) and is linked to serious health problems. Female wheelchair athletes are especially prone to LEA and seem to be at risk to develop RED-S symptoms (Egger and Flueck, 2020).
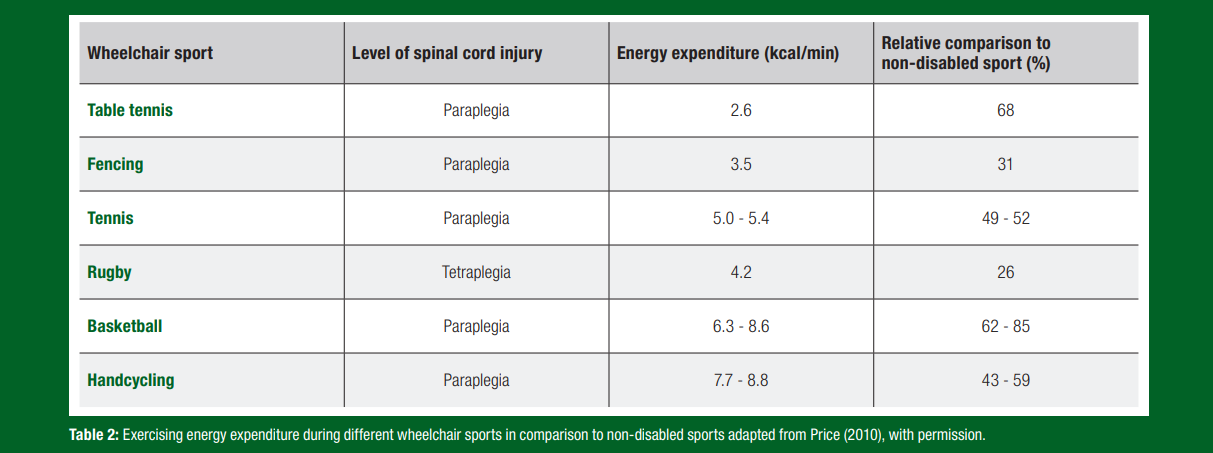
EE and AMP
Athletes with a limb deficiency exhibited a 19% lower TDEE compared to athletes with VI (3140 ± 837 kcal·d−1 vs. 3882 ± 1003 kcal·d−1), but a 32% higher value then athletes with SCI (2379 ± 522 kcal·d−1) (Weijer et al., 2024). However, the TDEE of athletes with AMP can vary dramatically depending on the etiology of the amputation (e.g., arm vs. leg; distal vs. proximal). In general, the more proximal an amputation the greater the EE during exercise. For example, using an above-knee prosthesis can result in 55-65% increases in oxygen consumption during exercise compared to non-disabled counterparts (Broad, 2019).
Interestingly, there appears to be a link between EE and the cause of amputation (e.g., peripheral vascular disease and diabetes vs. congenital causes or traumatic injury), with disease-related injuries leading to a 20-35% improvement in EE (Schmalz et al., 2002). Rough estimations of body proportional values and their contribution to energy requirements: head 8%; trunk 50%, total arm 5%, total leg 16%. These numbers might be used to correct RMR in athletes with AMP based on prediction equations (Broad, 2019). However, as these equations require height and body mass - which are not always easy to determine in athletes with AMP – their application is not recommended, especially as no data validation is available for this population. Thus, the use of indirect calorimetry to estimate RMR and EE during exercise is preferable whenever possible (Broad, 2019). From a practical perspective, rapid body mass gains or losses should be avoided in athletes with AMP, as these changes may affect the fit of the prosthesis, leading to discomfort and potential pressure sores.
EE and CP
Similar to the above-mentioned types of disability, energy requirements for this population are very heterogeneous, and TDEE is highly variable, influenced by factors such as sex, ambulation status, spasticity and body composition (Flueck, 2021). These individual circumstances must be considered when providing sports nutritional counselling and support to athletes with CP. Using predictive equations for RMR estimation seems to overestimate EE, and direct measurement is recommended whenever possible (Broad, 2019). RMR in athletes with CP was found to be around 26 ± 2.7 kcal·kg-1 FFM·day-1, comparable to Para athletes with VI and limb deficiency (Juziwak et al., 2016). However, data on energy demands of athletes with CP, especially during exercise, are missing and warrant further studies. According to Blauwet et al. (2017), athletes with CP are likely to be affected by LEA and related symptoms such as RED-S, highlighting the need for future research on energy requirements, EE and body composition in Para athletes with CP.
EE and VI
Athletes with VI are physiologically similar to non-disabled athletes and have reported RMR values of 1426 kcal·d−1 for males and 1387 kcal·d−1 for females, respectively (Juziwak et al., 2016). The TDEE for these athletes was reported to be (3140 ± 837 kcal·d−1), which appears similar to values observed in wheelchair tennis (3082 ± 381 kcal·d−1) (Weijer et al., 2024). Consequently, the primary challenges for sports nutrition practitioners working with athletes with VI are not related to determining precise energy needs and expenditure but rather to provide effective information and supporting athletes with VI in preparing their own menus, as well as selecting appropriate portion sizes and amounts of food.
PRACTICAL APPLICATIONS
• Tailored Energy Expenditure (EE) Monitoring: Regularly assess and monitor EE using individualized metrics, especially for para-athletes with complex needs like those with SCI or CP, as EE can vary significantly based on muscle mass, autonomic function and movement limitations.
• Customized Body Composition Assessment Techniques: Use advanced methods like DXA to accurately measure fat and lean mass, as standard techniques like BMI may not suit Para athletes with altered body fat distribution. A full skinfold thickness profile may be suitable to measure changes over time if done from an individual perspective.
• Optimized Movement Efficiency in CP and AMP Athletes: Develop training programs for CP and AMP athletes that focus on improving technique and strength to enhance stabilization and efficiency to reduce the elevated EE costs of movement.
• Nutritional Support for Athletes with VI: While energy requirements for athletes with VI are similar to those of non-disabled athletes, the primary challenges lie in providing accessible nutritional guidance, assisting with menu preparation and supporting portion size and food quantity selection.
• Regular Sports Nutrition Counselling: Given the complexity of EE and physiological needs in Para sport, athletes should receive consistent guidance tailored to their specific impairment and performance goals.
SUMMARY
This SSE article highlights the unique physiological and nutritional considerations for Para athletes across impairment types, including SCI, AMP, CP and VI. In addition, this SSE discusses how factors such as reduced muscle mass, movement inefficiencies, thermoregulatory challenges and body composition variances influence EE and performance. Tailored assessment methods, individualized training programs and specific sports nutrition strategies are emphasized to address these challenges, optimize health and enhance athletic performance. The importance of consistent, impairment-specific nutritional counselling is understood to prevent issues like LEA and related health risks.
The views expressed are those of the author and do not necessarily reflect the position or policy of PepsiCo, Inc.
REFERENCES
Abel, T., M. Kröner, S. Rojas Vega, C. Peters, C. Klose, and P. Platen (2017). Energy expenditure in individuals with spinal cord injury: Validity of predictive equations. J. Spinal Cord Med. 40:766–776.
Bhambhani, Y. (2002). Physiology of wheelchair racing in athletes with spinal cord injury.
Sports Med. 32:23-51.
Blauwet, C.A., E.M. Brook, A.S. Tenforde, E. Broad, C.H. Hu, E. Abdu-Glass, and E.G. Matzkin (2017). Low energy availability, menstrual dysfunction, and low bone mineral density in individuals with a disability: Implications for the para athlete population. Sports Med. 47:1697-1708.
Broad, E. (2019). Sports Nutrition for Paralympic Athletes. Boca Raton (FL): CRC Press.
Brown, R., A.F. DiMarco, J.D Hoit, and E. Garshick (2006). Respiratory dysfunction and management in spinal cord injury. Respir. Care. 51:853-870.
Crandall, C.G., and S.L. Davis (2010). Cutaneous vascular and sudomotor responses in human skin grafts. J. Appl. Physiol. 109:1524-1530.
Croft, L., S. Dybrus, J. Lenton, and V. Goosey-Tolfrey (2010). A comparison of the physiological demands of wheelchair basketball and wheelchair tennis. Int. J. Sports Physiol. Perform. 5:301-315.
Egger, T., and J.L. Flueck (2020). Energy availability in male and female elite wheelchair athletes over seven consecutive training days. Nutrients. 12:3262.
Flueck, J. (2020). Body composition in Swiss elite wheelchair athletes. Front. Nutr. 7:1. Flueck, J.L (2021). Nutritional considerations for Para-cycling athletes: A narrative review.
Sports (Basel). 9:154.
Flueck, J.L., and J.A. Parnell (2021). Protein considerations for athletes with a spinal cord injury. Front. Nutr. 8:652441.
Goosey-Tolfrey, V.L., and Leicht, C.A. (2013). Field-based physiological testing of wheelchair athletes. Sports Med. 43:77-91.
Goosey-Tolfrey, V.L., and O’Brien, T.J. (2024). Thermoregulatory challenges in Para-sport: Current perspectives. SSE #259. Available at: https://www.gssiweb.org/en/sports- science-exchange/Article/thermoregulatory-challenges-in-para-sport-current- perspectives.
Grossmann, F., J.L. Flueck, C. Perret, R. Meeusen, and B. Roelands (2021). The thermoregulatory and thermal responses of individuals with a spinal cord injury during exercise, acclimation and by using cooling strategies - A systematic review. Front. Physiol. 12:636997.
Hopman, M.T., B. Oeseburg, and R.A. Binkhorst (1992). Cardiovascular responses in paraplegic subjects during arm exercise. Eur. J. Appl. Physiol. 65:73-78.
Iturricastillo, A., C. Granados, J. Yanci, A. Badiola, and L. Olarte (2016). Peripheral fatigue and muscle activity after repeated sprints in wheelchair basketball players. Int. J. Sports Physiol. Perform. 11:245–252.
Juziwak, C.R., C. Winckler, D.P. Joaquim, A. Silva, and M.T. de Mello (2016). Comparison of measured and predictive values of basal metabolic rate in Brazilian Paralympic track and field athletes. Int. J. Sport Nutr. Exerc. Metab. 26:330-337.
Kloyiam, S., S. Breen, P. Jakeman, J. Conway, and Y. Hutzler (2011). Soccer-specific endurance and running economy in soccer players with cerebral palsy. Adapt. Phys. Activ. Q. 28:354-367.
Klute, G.K., G.I. Rowe, A.V. Mamishev, and W.R. Ledoux (2007). The thermal conductivity of prosthetic sockets and liners. Prosthet. Orthot. Int. 31:292-299.
Magno e Silva, M.P., C. Winckler, A.A. Costa e Silva, J. Bilzon, and E. Duarte (2013). Sports injuries in Paralympic track and field athletes with visual impairment. Med. Sci. Sports Exerc. 45:908-913.
Maltais, D., B. Wilk, V. Unnithan, and O. Bar-Or (2004). Responses of children with cerebral palsy to treadmill walking exercise in the heat. Med. Sci. Sports Exerc. 36:1674-1681. Mengelkoch, L.J., J.T. Kahle, and M.J. Highsmith (2017). Energy costs and performance of transfemoral amputees and non-amputees during walking and running: A pilot study.
Prosthet. Orthot. Int. 41:484-491.
Nash, M.S. (2000). Known and plausible modulators of depressed immune functions following spinal cord injuries. J. Spinal Cord Med. 23:109-110.
O'Brien, T.J., K.M Lunt, B.T. Stephenson, and V.L. Goosey-Tolfrey (2022). The effect of pre- cooling or per-cooling in athletes with a spinal cord injury: A systematic review and meta-analysis. J. Sci. Med. Sport. 25:606-614.
Paulson, T.A.W., and V.L. Goosey-Tolfrey (2017). Current perspectives on profiling and enhancing wheelchair court sport performance. Int. J. Sports Physiol. Perform. 12:275-286.
Price, M. (2010). Energy expenditure and metabolism during exercise in persons with a spinal cord injury. Sports Med. 40:681–696.
Ruettimann, B., C. Perret, J.A. Parnell, and J.L. Flueck (2021). Carbohydrate considerations for athletes with a spinal cord injury. Nutrients. 13:2177.
Scaramella, J., S.D. Tagliaferri, C.M. Prado, and Z.M. Ferraro (2017). Dietary intake and body composition in athletes with a physical disability: A systematic review. Sports Med. 47:2151-2160.
Schmalz, T., S. Blumentritt, and R. Jarasch (2002). Energy expenditure and biochemical characteristics of lower limb amputee gait: The influence of prosthetic alignment and different prosthetic components. Gait Posture. 16: 255-263.
Schmid, A., M. Huonker, P. Stober, J. Keul, and W. Kindermann (1998). Catecholamine response of individuals with spinal cord injury during exercise and recovery. Spinal Cord. 36:290–297.
Sherk, V. D., M.G. Bemben, and D.A. Bemben (2010). Interlimb muscle and fat comparisons in persons with lower-limb amputation. Arch. Phys. Med. 91:1077-1081.
Slater, G. and V.L. Goosey-Tolfrey (2019). Body Composition. In Sports Nutrition for Paralympic Athletes. E. Broad (ed.). Boca Raton (FL): CRC Press. pp. 245-264.
Steinberg, L.L., F.A. Lauro, D.R. Almeida, M.B. Bouzas, L. Carnaz, and A. Cliquet (2000). Alterations in heart rate variability in quadriplegic and paraplegic subjects. Braz. J. Med. Biol. Res. 33:1009-1014.
Stephenson, B., and V.L. Goosey-Tolfrey (2020). Physiological considerations for paratriathlon training and competition. In Triathlon Medicine. S. Migliorini (ed.). Springer Nature Switzerland. pp. 393-415.
Sutton, L., J. Wallace, V. Goosey-Tolfrey, M. Scott, and T. Reilly (2009). Body composition of female wheelchair athletes. Int. J. Sports Med. 30:259-265.
Taylor, J.B., G. Santi, and S.D. Mellalieu (2016). Freestyle race pacing strategies (400 m) of elite able-bodied swimmers and swimmers with disability at major international championships. J. Sports Sci. 34:1913-1920.
Ward, K.H., and M.C. Meyers (1995). Exercise performance of lower-extremity amputees.
Sports Med. 20:207-214.
Webborn, A.D.J. (1996). Heat-related problems for the Paralympic Games, Atlanta. Br. J. Ther. Rehabil. 3:429-436.
Webborn, N. and P. Van de Vliet (2012). Paralympic medicine. Lancet 380:65-71.
Weijer, V.C.R., K.L. Jonvik, L. Van Dam, L. Risvang, G. Plasqui, Ø Sandbakk, T. Raastad,
L.J.C. Van Loon, and J.W. Van Dijk (2024). Energy requirements of Paralympic athletes: Insights from the doubly labeled water approach. Med. Sci. Sports Exerc. 56:963-9